Research
Introduction to matter-wave atom interferometry
In quantum metrology, atom interferometry is used to measure important properties of our Universe with the highest precision: the gravitational constant, the fine-structure constant, deviations from Newtonian gravity and general relativity. In addition, it has serious practical applications such as gravimetry (measuring Earth’s gravity), inertial sensing, and probing geodesy and geophysics. But what is an atom interferometer and how does it work?
Atom interferometers capitalize on the fact that atoms, just like light, are waves. In a light interferometer (imagine the now-famous LIGO interferometer, for example), we use glass or crystals to split, redirect and recombine the laser beam onto multiple paths. The interferometer signal is then read out on a photodetector, providing exceedingly accurate information about the difference in the propagation of the light waves along the different paths.
In analogy, atom interferometers use laser beams that act as effective diffraction gratings to split, redirect and recombine atomic wavepackets. Quantum mechanics allows a single atom to be separated into wavepackets that can then travel along different paths, a state sometimes referred to as “quantum spatial superposition”. Unlike photons, atoms are massive, which makes them directly sensitive to both gravitational and inertial effects due to Einstein’s equivalence principle.
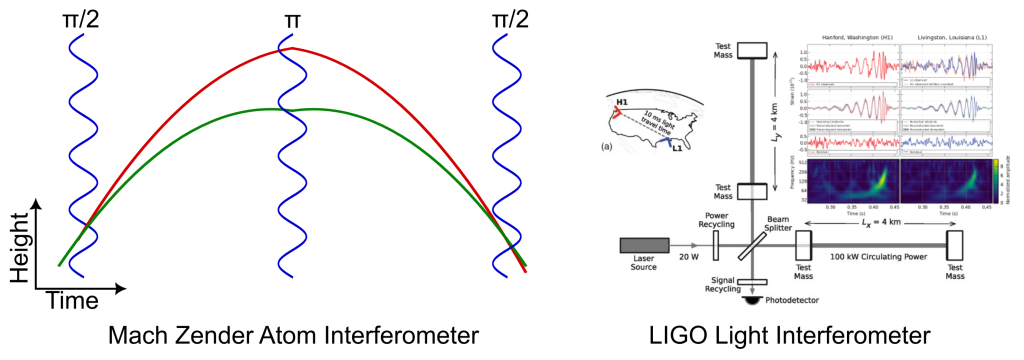
The frequency (periodicity) of the laser is supremely well controlled through methods ubiquitous in AMO (atomic, molecular and optical) physics. These methods often make use of the intrinsic resolving power and stability of optical transitions in an atom, such as in atomic clocks. This stability enables measurements with paramount accuracy.
Interferometry with atoms suspended in an optical lattice
Atom interferometers traditionally use atomic fountains, where atoms in free-fall inside ultra-high vacuum chambers are well-insulated from the environment to avoid decoherence of the fragile atomic quantum superposition state. However, using atoms in free-fall in a meters-long apparatus limits measurement time to a few seconds and much less when measuring interactions with a small source mass.
Recently, interferometers with atoms suspended for 70 seconds in an optical lattice have been demonstrated. However, the optical lattice must balance Earth’s gravity by applying forces that are a billion-fold stronger than the signals of interest. The effect of these strong interactions on the fragile quantum spatial superposition state must be well-understood and kept under a high degree of control. To this end, we minimize imperfections in the optical lattice by mode-filtering with an optical cavity.
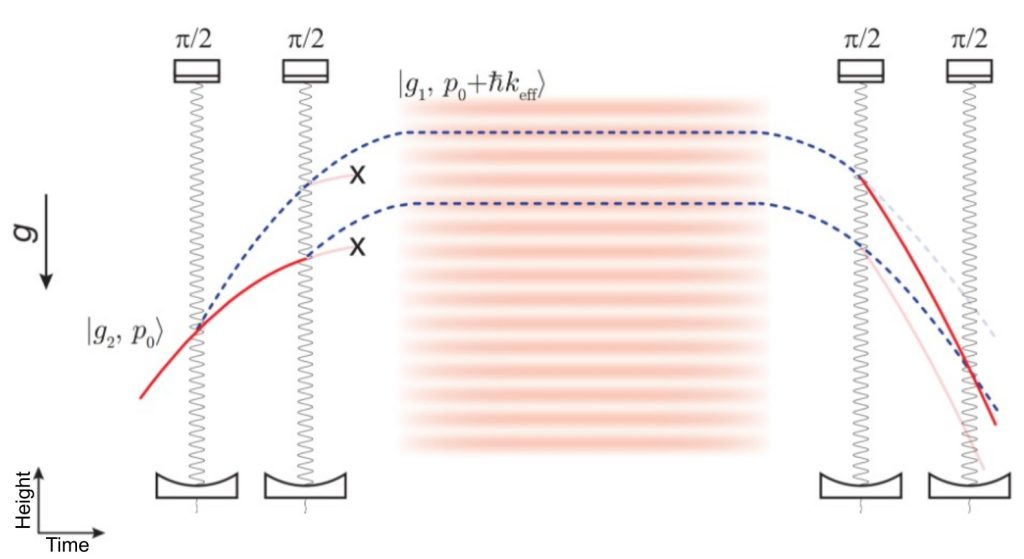
Our work focuses on improving the sensitivity of lattice atom interferometry, exploring new methods to probe physics beyond the Standard Model and also developing practical applications in a real-world setting. For more details, see the individual pages for our two primary research directions: